List of members
Facilities
Internships and jobs
PhD
Publications
News
Team
- Permanent members: Catherine Gourdon, Laura Thevenard, Max Marangolo
- PhD (2021-2024) : Ashwin Vythelingum
- Post-doc (2023-2024): Djoudi Ourdani
In ferromagnetic metallic or semiconductor materials, we have developed different approaches to manipulate magnetization in a non-inductive way, exploiting in particular the magneto-elastic coupling with acoustic waves (strain waves). Their wave nature and low attenuation open interesting perspectives for magnonics (“2019 Surface Acoustic Wave Roadmap“).
We investigated in detail the diluted magnetic semiconductors (Ga,Mn)As and (Ga,Mn)(As,P) (epitaxy at C2N by A. Lemaître), key materials in spin electronics as they could address both the “logic” and “memory” aspects of a device. The main results are:
- Magneto-elastic control of magnetization by surface acoustic waves (SAWs):
- SAW-induced ferromagnetic resonance [PRB14],[JPhysConMat18], [PRApp18],
- SAW-induced resonant and non-resonant magnetization switching, [PRB13], [PRB16prec], [JPhysConMat18], [PRevApp19], [PRB16nuc]
- Magnetization control by femtosecond laser pulses:
- Dynamics of domain walls: [PRB17-dwp], [PRB13DW], [PRB12], [PRB11]
By capitalizing on the experience acquired in magneto-acoustics, we now seek to understand if it is possible
- to manipulate the static or dynamic states of antiferromagnets by acoustic waves by taking advantage of their magnetostriction (ANR ACAF project)
- or vice versa to use the particular properties of antiferromagnets to improve surface acoustic wave RF devices (ANR MAXSAW project)
- to generate acoustically and propagate spin waves in Fe/FeN pads and conduits (PEPR SPIN, SWING project).
Main experimental techniques
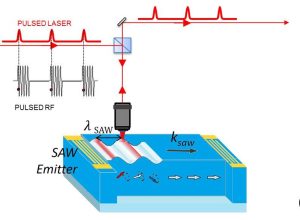
Schematics of the experimental setup showing the excitation and detection of surface acoustic waves by interdigited transducers and synchronized optical detection of the magnetization dynamics by laser pulses.
- Surface acoustic waves, with the Acoustics and optics for Nanosciences and quantum physics team
- Kerr effect microscopy (polar and longitudinal configurations)
- Time-resolved Kerr effect (pump-probe)
Collaborations
- J. A. Arregi, V. Uhlíř, CEITEC BUT, Brno University of Technology, Czech Republic
- M. LoBue, M. Almanza, A. Pecheux, Université Paris-Saclay, ENS Paris-Saclay, CNRS, SATIE, France
- A. Castellano, M. J. Verstraete, NanoMat/Q-Mat, Université de Liège, and European Theoretical Spectroscopy Facility, Belgium
- R. Lopes Seeger, F. Millo, C. Chappert, and T. Devolder, Université Paris-Saclay, CNRS, Centre de Nanosciences et de Nanotechnologies, Palaiseau, France
- A. Solignac, G. de Loubens, SPEC, CEA, CNRS, Université Paris-Saclay, France
- A. Bartasyte, S. Margueron, L. La Spina, V. Laude, Université de Franche-Comté, CNRS, Institut FEMTO-ST, Besançon, France
- J. Sampaio, A. Mougin, Laboratoire de Physique des solides, Université Paris-Saclay, CNRS, Orsay, France
- Y. Roussigné, M. Belmeguenai, Laboratoire des Sciences des Procédés et des Matériaux, Université Paris 13, Sorbonne Paris Cité, Villetaneuse, France
Funding
- French National Research Agency (ANR ACAF 20-CE30-0027)
- French National Research Agency (ANR MAXSAW-20-CE24-0025)
- France 2030 program – PEPR SPIN, Project SWING (ANR 22 EXSP 0004)
Publications
- [Preprint] Magnetic phase dependency of the thermal conductivity of FeRh from thermoreflectance experiments and numerical simulations, Aloïs Castellano, Kévin Alhada-Lahbabi, Jon Ander Arregi, Vojtěch Uhlíř, Bernard Perrin, Catherine Gourdon, Daniele Fournier, Matthieu Jean Verstraete, L. Thevenard (preprint), https://hal.science/hal-04531324
- [Preprint] Experimental determination of the temperature-and phase-dependent elastic constants of FeRh, D. Ourdani, A. Castellano, A. K. Vythelingum, J. A. Arregi, V. Uhlíř, B. Perrin, M. Belmeguenai, Y. Roussigné, C. Gourdon, M.J. Verstraete, and L. Thevenard (preprint), https://cnrs.hal.science/hal-04526444
- [PRB24] Symmetry of the coupling between surface acoustic waves and spinwaves in synthetic antiferromagnets, R. Lopes Seeger, L. La Spina, V. Laude, F. Millo, A. Bartasyte, S. Margueron, A. Solignac, G. de Loubens, L. Thevenard, C. Gourdon, C. Chappert, and T. Devolder, Phys. Rev. B 109, 104416 (2024),
- [PRB20] Time- and space-resolved nonlinear magnetoacoustic dynamics, M. Kraimia, P. Kuszewski, J.-Y. Duquesne, A. Lemaître, F. Margaillan, C. Gourdon, and L. Thevenard Phys. Rev. B 101, 144425 (2020)
- [JAP20] Exploring the shear strain contribution to the uniaxial magnetic anisotropy of (Ga,Mn)As, M. Kraimia, L. Largeau, K. Boujdaria, B. Croset, C. Mocuta, A. Lemaître, C. Gourdon, and L. ThevenardJournal of Applied Physics 127, 093901 (2020)
- [JPhysD19] The 2019 surface acoustic waves roadmap, P. Delsing, C. Gourdon, L. Thevenard et al. J. Phys. D : Applied Physics 52, 353001 (2019)
- [PRevApp19] Field-Free Magnetization Switching by an Acoustic Wave, I. S. Camara, J.-Y. Duquesne, A. Lemaître, C. Gourdon, L. Thevenard, Phys. Rev. Applied 11, 014045 (2019)
- [PRApp18] Optical Probing of Rayleigh Wave Driven Magnetoacoustic Resonance, P. Kuszewski, J.-Y. Duquesne, L. Becerra, A. Lemaître, S. Vincent, S. Majrab, F. Margaillan, C. Gourdon, and L. Thevenard,Phys. Rev. Applied 10, 034036 (2018)
- [JPhysConMat18] Resonant magneto-acoustic switching : influence of Rayleigh wave frequency and wavevector, P. Kuszewski, I. S. Camara, N. Biarrotte, L. Becerra, J. von Bardeleben, W Savero Torres, A. Lemaître, C. Gourdon, J.-Y Duquesne, Journal of Physics : Condensed Matter 30 244003 (2018)
- [PRB17-sw] Counter-rotating standing spin-waves : a magneto-optical illusion , S. Shihab, L. Thevenard, A. Lemaître, Catherine Gourdon, Physical Review B 95 144411 (2017)
- [PRB17-dwp] Spin transfer and spin-orbit torques in in-plane magnetized (Ga,Mn)As tracks, L. Thevenard, B. Boutigny, N. Güsken, L. Becerra, C. Ulysse, S. Shihab, A. Lemaître, J.-V. Kim, V. Jeudy, C. Gourdon, Physical Review B 95 054422 (2017)
- [PRB17-soliton] Acoustic solitons : A robust tool to investigate the generation and the detection of ultrafast acoustic waves, E. Péronne, N. Chuecos, L. Thevenard, and Bernard Perrin, Physical Review B 95 064306 (2017)
- [JAP17] Vector network analyzer measurement of the amplitude of an electrically excited surface acoustic wave and validation by x-ray diffraction, I. Camara, B. Croset, L. Largeau, P. Rovillain, L. Thevenard, J.-Y. Duquesne, Journal of Applied Physics 121 044503 (2017)
- [JAC16] Laboratory X-ray characterization of a surface acoustic wave on GaAs : the critical role of instrumental convolution, L. Largeau, I. Camara, J.-Y. Duquesne, C. Gourdon, P. Rovillain, L. Thevenard, B. Croset, Journal of Applied Crystallography 49 2073 (2016)
- [PRB16prec] Precessional magnetization switching induced by a surface acoustic wave, L. Thevenard, I. S. Camara,S. Majrab, M. Bernard, P. Rovillain, A. Lemaître, C. Gourdon, and J.-Y. Duquesne, Physical Review B 93 134430 (2016)
- [JAP16] Stationary thermal gradient induced by ultrafast laser excitation in a ferromagnetic layer, S. Shihab, L. Thevenard, A. Lemaître, C. Gourdon, J.-Y. Duquesne J. Appl. Phys. 119 153904 (2016)
- [PRB16nuc] Strong reduction of the coercivity by a surface acoustic wave in an out-of-plane magnetized epilayer, L. Thevenard, I. S. Camara, J.-Y. Prieur, P. Rovillain, A. Lemaître, C. Gourdon, and J.-Y. Duquesne, Physical Review B 93, 140405(2016)
- [JMMM15] Optimizing magneto-optical effects in the ferromagnetic semiconductor GaMnAs, H. Riahi, L. Thevenard, M. Maaref, B. Gallas, A. Lemaître, C. Gourdon, Journal of Magnetism and Magnetic Materials 395, 340 (2015)
- [APL15] Systematic study of the spin stiffness dependence on Phosphorus alloying in the ferromagnetic semiconductor (Ga,Mn)As , S. Shihab, H. Riahi, L. Thevenard, H. J. Von Bardeleben, A. Lemaître, C. Gourdon, Appl. Phys. Lett. 106 142408 (2015)
- [PRB14] Surface-acoustic-wave-driven ferromagnetic resonance in (Ga,Mn)(As,P) epilayers, L. Thevenard, C. Gourdon, J.Y. Prieur, H. J. von Bardeleben, S. Vincent, L. Becerra, L. Largeau, J.Y. Duquesne, Physical Review B 90, 094401 (2014)
- [PRB13] Irreversible magnetization switching using surface acoustic waves, L. Thevenard, J.-Y. Duquesne, E. Peronne, H. J. von Bardeleben, H. Jaffres, S. Ruttala, J-M. George, A. Lemaître, and C. Gourdon, Physical Review B 87, 144402 (2013)
- [JMMM13] Annealing effect on the magnetization reversal and Curie temperature in a GaMnAs layer, H. Riahi, W. Ouerghui, L. Thevenard, C. Gourdon, M.A. Maaref, A. Lemaître, O. Mauguin, C. Testelin, J. Magn. Mag. Mat. 342, 149 (2013)
- [PRB13DW] Domain-wall flexing instability and propagation in thin ferromagnetic films, C. Gourdon, L. Thevenard, and S. Haghgoo, A. Cebers, Phys. Rev. B 88, 014428 (2013)
- [JPhys13] The influence of phosphorus content on magnetic anisotropy in ferromagnetic (Ga, Mn)(As,P)/GaAs thin films , M Yahyaoui, K Boujdaria, M Cubukcu, C Testelin and C Gourdon, J. Phys. : Condens. Matter 25 346001 (2013)
- [APL12] Fast domain wall dynamics in MnAs / GaAs films Fast domain wall dynamics in MnAs / GaAs films, M. Tortarolo, L. Thevenard, H. J. von Bardeleben, M. Cubukcu, M. Eddrief, V. Etgens, C. Gourdon, Applied Physics Letters 101, 072408 (2012)
- [PRB12] High domain wall velocities in in-plane magnetized (Ga,Mn)(As,P) layers, Thevenard, L., Hussain, S. von Bardeleben, H. Bernard, M. Lemaître, A. Gourdon, C., Physical Review B 85 064419 (2012)
- [JAP12] The influence of the epitaxial strain on the magnetic anisotropy in ferromagnetic (Ga,Mn)(As,P)/GaAs thin films , M Yahyaoui, K Boujdaria, M Cubukcu, C Testelin and C Gourdon, J. App. Phys. 111 346001 (2012)
- [PRB11] Domain wall propagation in ferromagnetic semiconductors : Beyond the one-dimensional model, L. Thevenard, C. Gourdon, S. Haghgoo, J-P. Adam, J. von Berdeleben, A. Lemaître, W. Schoch, A. Thiaville, Physical Review B 83, 245211 (2011)
- [PRB10] Effect of picosecond strain pulses on thin layers of the ferromagnetic semiconductor (Ga,Mn)(As,P), L. Thevenard, E. Peronne, C. Gourdon, C. Testelin, M. Cubukcu, E. Charron, S. Vincent, A. Lemaître, and B. Perrin, Phys. Rev. B 82, 104422 (2010)
- [PRB10_] Exchange constant and domain wall width in (Ga,Mn)(As,P) films with self-organization of magnetic domains, S. Haghgoo, M. Cubukcu, H. J. von Bardeleben, L. Thevenard, A. Lemaître, and C. Gourdon, Phys. Rev. B 82, 041301 (2010)
- [PRB09] Unusual domain-wall motion in ferromagnetic semiconductor films with tetragonal anisotropy, C. Gourdon, V. Jeudy, A. Cēbers, A. Dourlat, Kh. Khazen, and A. Lemaître, Phys. Rev. B 80, 161202(R) (2009).
- [PRB08] Field-Driven Domain Wall Dynamics in GaMnAs Films with Perpendicular Anisotropy, A. Dourlat, V. Jeudy, A. Lemaître, and C. Gourdon, Phys. Rev. B 78, 161303(R) (2008).
- [Lemaître08] Strain control of the magnetic anisotropy in (Ga,M n) (As,P) ferromagnetic semiconductor layers, A. Lemaître, A. Miard, L. Travers, O. Mauguin, L. Largeau, C. Gourdon, V. Jeudy, M. Tran, and J.-M. George, Appl. Phys. Lett. 93, 021123 (2008).
- [PRB07] Determination of the micromagnetic parameters in GaMnAs using domain theory, C. Gourdon, A. Dourlat, V. Jeudy, K. Khazen, H. J. von Bardeleben, L. Thevenard, and A. Lemaître, Phys. Rev. B 76, 241301(R) (2007).
- [Dourlat07] Domain structure and magnetic anisotropy fluctuations in (Ga,Mn)As : Effect of annealing, A. Dourlat, V. Jeudy, C. Testelin, F. Bernardot, K. Khazen, C. Gourdon, L. Thevenard, L. Largeau, O. Mauguin, and A. Lemaître, J. Appl. Phys. 102, 023913 (2007).
- [Dourlat08] Experimental determination of domain wall width and spin stiffness constant in ferromagnetic (Ga,Mn)As with perpendicular easy axis A. Dourlat, C. Gourdon, V. Jeudy,, K. Khazen, H.J. von Bardeleben, L. Thevenard, A. Lemaitre, Physica E 40 (2008) 1848–1850
- [Dourlat07] Domain wall dynamics in annealed GaMnAs epilayers A. Dourlat, V. Jeudy, L. Thevenard, A. Lemaître, and C. Gourdon, J. Supercond. Nov. Magn. 20, 453 (2007).
- [Dourlat07] Expansion and collapse of domains with reverse magnetization in GaMnAs epilayers with perpendicular magnetic easy axis A. Dourlat, C. Gourdon, V. Jeudy, C. Testelin, K. Khazen, J.L. Cantin, H.J. von Bardeleben, L. Thevenard, A. Lemaitre, IEEE Trans. Magn. 43, 3022 (2007).